Ice pellets, graupel, and freezing drizzles! Oh my!
Some winter storms bring glorious heaps of snow. Some instead bring ever-annoying sleet. Still others cause terrible downpours of freezing rain, leaving centimetres of ice and trails of destruction in their wake. Understanding and predicting the type of precipitation that falls when the air is near freezing is crucial for both human and natural systems. Yet, it remains one of the toughest challenges in meteorology today.
A year ago, we wrote an article about WINTRE-MIX — a ground-breaking experiment conducted in part at the Gault Nature Reserve. The international team of researchers behind it was seeking to explain why some winter storms offer a good day of skiing while others cause downed powerlines and states of emergency. Their first results were recently published, providing one of the clearest pictures to date of winter storms and the precipitation they bring.
More than just rain vs. snow
When temperatures in the atmosphere are consistently above or below zero degrees Celsius, precipitation types are mostly predictable. For instance, snow falls when the air mass above you is below freezing. And when it is above freezing, it rains. We even have weather forecasts and physics models that describe how much precipitation and which type will fall.
Things get complicated, however, when temperatures change from above to below freezing with altitude. Under these conditions, snowflakes at the top of clouds melt, refreeze and interact with one another as they fall. Depending on the temperature gradients and kinds of interactions that occur on their journey to the ground, these snowflakes transform into a wintery mix of snow, rain, sleet, freezing rain, ice crystals, and graupel.
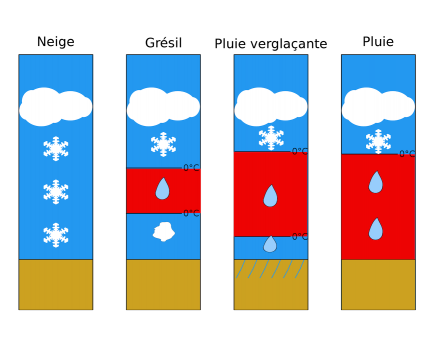
A simplified diagram showing the temperature gradients that cause (from left to right) snow, ice pellets, freezing rain, and rain (photo: Minder et al. 2023)
Freezing rain, for example, begins when snow falls through a layer of the atmosphere at temperatures above zero and turns into rain. These raindrops continue falling like a liquid until they hit a shallow, sub-zero layer of air just above the ground. The rain does not have time to turn back into ice before it hits the surface and thus freezes on contact — resulting in the accumulation of solid ice on trees, powerlines, and roads.
Each near-zero precipitation type has its own unique properties, conditions for formation, and implications for people and ecosystems on the ground. These near-zero precipitation types are especially sensitive to their surroundings. According to the WINTRE-MIX researchers, they are “strongly sensitive to variations in temperature as small as ±0.5°C”, often at heights that are hard to measure by traditional meteorological methods. In turn, predicting near-zero precipitation types remains “the most serious problem in wintertime … forecasting.”
The methods of WINTRE-MIX
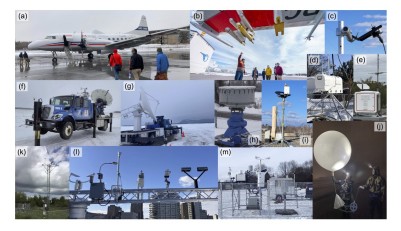
Examples of some of the radar, ground-based, and airborne technology used in the WINTRE-MIX experiment (photo: Minder et al. 2023)
To help resolve these predictability challenges, the WINTRE-MIX team devised a comprehensive and ambitious experiment across the St. Lawrence River and Champlain valleys. The scientists from 9 different research institutes across Canada and the United States set up research stations in New York, Vermont, Ontario, and Quebec (including one here at Gault) during the winter of 2022. With each passing winter storm, the stations employed various sampling methods to capture what was happening in the atmosphere across the landscape.
The scientists examined factors as small as an individual raindrop and as big as the entire St. Lawrence River valley. For example, the team deployed sensors on the ground at Gault and mounted on an aircraft to quantify minuscule factors like the size and shape of individual ice crystals. Meanwhile, radars were observing the intensity of precipitation across the entirety of the St. Lawrence River valley. At the same time, synchronized launches of weather balloons were taking temperature, air pressure, and wind speed readings of the entire atmosphere. Thus, they could capture unprecedentedly detailed observations about why different types of winter precipitation form.
New insights
In this first publication, the researchers used the multiscale data they collected to describe one particularly complex storm from six different perspectives. Using the winter storm of February 23rd, 2022, as a case study, the scientists used regional, local, and microscopic observations to explain the factors that correlate with different kinds of winter precipitation.
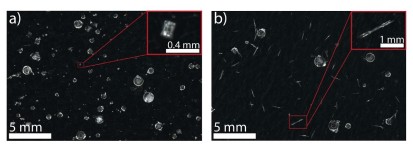
Two macro photographs taken two hours apart during the Feb 22nd storm at the research station in Sorel, QC. Different secondary crystal structures occurred with larger ice pellets at the site, with column crystals in photo A and longer needle crystals in photo B (photo: Minder et al. 2023)
The researchers noted several unique phenomena across these overlapping scales of analysis.
From the ground stations, weather balloons, and radar measurements, they found areas of unexpected refreezing. Typical weather models for these locations predicted freezing rain; however, fully frozen ice pellets fell instead. The paper suggests that secondary ice crystals, like those observed in photos a and b, may have sped up this transformation.
The team also observed terrain-modified air flows from their doppler radars and weather balloons. These cold surface winds moved up the valley due to the surrounding topography, against the general wind pattern. These winds brought with them sub-zero temperatures that modified precipitation, in some cases contributing to the rapid refreezing they noted on the ground. At different times during the storm, these winds varied in intensity and location- causing shifting mixes of sleet and freezing rain across the valley.
For the researchers, the phenomena observed through their multiscale methodology brought as many new questions as answers. Why did this sudden refreezing occur during this storm? How did secondary ice crystals play in this ice pellet formation? Why did terrain-modified flows persist in some places and not in others? How can we use these observations to make weather forecasts better?
Thanks to this first paper, scientists are one storm closer to fully grasping the complexity of near-freezing precipitation. And as with all good things in science, the WINTRE-MIX experiment has inspired many new research questions. Best of all, it has provided a season’s worth of high-quality, open-source data with which to investigate them.
About the researchers
The WINTRE-MIX project is led by American researchers from University of Albany (Dr. Justin Minder and Dr. Nick Bassill), University of Wyoming (Dr. Jeffrey R. French and Dr. David Kingsmill), University of Colorado (Dr. Katja Friedrich, Dr. Andrew Winters), and the National Research Council (Dr. Leonid Nichman, Dr. Cuong Nguyen).
They collaborated with local scientists Dr. John Gyakum, Dr. Frédéric Fabry and Dr. Daniel Kirshbaum from the Atmospheric and Oceanic Sciences department of McGill University and Dr. Julie M. Thériault from UQAM (Université du Québec à Montréal).
Learn more about the WINTRE-MIX project by visiting their website or follow them on Twitter to get updates.
About the article
Minder, J. R., Bassill, N., Fabry, F., French, J. R., Friedrich, K., Gultepe, I., Gyakum, J., Kingsmill, D. E., Kosiba, K., Lachapelle, M., Michelson, D., Nichman, L., Nguyen, C., Thériault, J. M., Winters, A. C., Wolde, M., & Wurman, J. (2023). P-type Processes and Predictability: The Winter Precipitation Type Research Multiscale Experiment (WINTRE-MIX). Bulletin of the American Meteorological Society, 1(aop). https://doi.org/10.1175/BAMS-D-22-0095.1
Greg Roberts
Field Operations Assistant
Gault Nature Reserve of McGill University
Header: Researchers Karel Veilleux (left) and Juliann Wray (right) are ready to launch their first meteorological sounding system of the night (photo: Alex Tran)